20.109(F20):M3D3
Introduction
The CRISPRi system involves three genetic components: the pdCas9 plasmid (1 in image below), the psgRNA_target plasmid (2 in image below), and the targeted gene within the host genome (3 in image below). Though the targeted gene is native to the host genome, the plasmids must be transformed into the cell and maintained using antibiotic selection. Thus far in this module, we have discussed the CRISPRi plasmids as individual units, but now we will consider the system as a whole in the context of engineering gene expression.
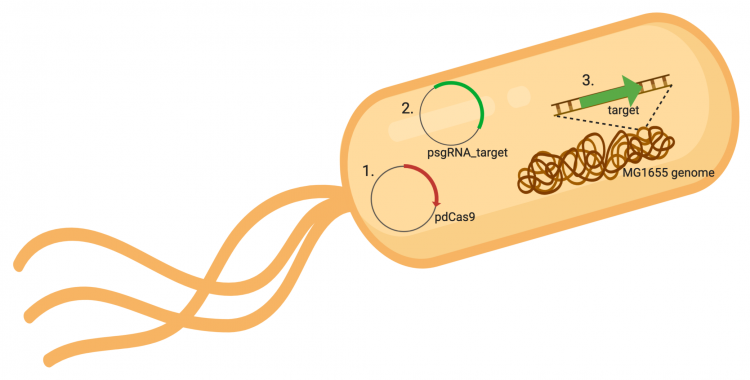
In the previous laboratory session, you reviewed the procedure used to generate the psgRNA_target plasmids. Today we will discuss how the CRISPRi system (pdCasd9 and psgRNA_target) is transformed into E. coli and, once transformed into the bacterial cells, how the psgRNA_target and dCas9 are transcribed from the expression plasmids. Briefly, the promoter (pJ23119) driving expression of the sgRNA sequence in the gRNA_target plasmid is constitutively active. This means that transcription of the gRNA sequence specific to the target in the host genome is constitutive. Therefore, your sgRNA_target is always present in the MG1655 cells.
In contrast, expression of the gene encoding dCas9 within pdCas9 is regulated by an inducible promoter (pLtetO-1). An inducible promoter is 'off' unless the appropriate molecule is present to relieve repression. In the case of the CRISPRi system, expression of the gene encoding dCas9 is inhibited due to the use of a tet-based promoter construct. Tet is shorthand for tetracycline, which is an antibiotic that inhibits protein synthesis through preventing the association between charged aminoacyl-tRNA molecules and the A site of ribosomes. Bacterial cells that carry the tet resistance cassette are able to survive exposure to tetracycline by expressing genes that encode an efflux pump that 'flushes' the antibiotic from the bacterial cell. To conserve energy, the tet system is only expressed in the presence of tetracyline. In the absence of tetracycline, a transcription repressor protein (TetR) is bound to the promoter upstream of the tet resistance cassette genes. When tetracycline is present, the molecule binds to TetR causing a confirmational change that results in TetR 'falling off' of the promoter. In the CRISPRi system, the tet-based promoter construct upstream of the gene that encodes dCas9 is 'off' unless anhydrotetracyline (aTc), an analog of tetracyline, is added to the culture media. Why is it important to use an analog rather than the actual antibiotic?Taken together, the sgRNA_target is constitutively transcribed and, as stated above, always present. The dCas9 protein is only present when aTc is added. Thus, gene expression is only altered when aTc is present. In this, when dCas9 is expressed it forms a complex with the sgRNA_target. The sgRNA target then 'seeks out' the target within the host genome. When the targeted sequence is recognized, the complex binds and acts as a 'roadblock' by prohibiting RNAP access to the sequence. Because the targeted gene is not able to be transcribed, the protein encoded by that gene is not synthesized. In our experiments, we hypothesize that the absence of specific proteins, or enzymes, involved in anaerobic fermentative metabolism will increase the yield of ethanol.
Protocols
Part 1: Review experimental setup
DISCUSS PROTOCOLS BRIEFLY RATHER THAN STEP BY STEP
Transform CRISPRi system into MG1655 E. coli cells
During “transformation,” a plasmid enters a bacterium and, once inside, replicates and expresses the genes it encodes. In our case, the goal is for each plasmid to enter a bacterium in a method referred to as co-transformation. As mentioned above, a gene on the pgRNA plasmid leads to ampicillin-resistance and a gene on the pdCas9 plasmid leads to chloramphenicol-resistance. Thus, only a co-transformed bacterium will grow on agar medium containing the antibiotics ampicillin and chloramphenicol. Untransformed cells will die before they can form a colony on the agar surface.
Most bacteria do not usually exist in a “transformation ready” state, but the bacteria can be prepared such that they are permeable to the plasmid DNA. Cells that are capable of transformation are referred to as “competent.” Competent cells are extremely fragile and should be handled gently, specifically kept cold and not vortexed. The transformation procedure is efficient enough for most lab purposes, with efficiencies as high as 109 transformed cells per microgram of DNA, but it is important to realize that even with high efficiency cells only 1 DNA molecule in about 10,000 is successfully transformed.You will transform your pgRNA plasmids with the pdCas9 plasmid into E. coli MG1655, which is the strain we will use to examine the effect of your approach on either ethanol or acetate production.
- Label two 1.5 mL eppendorf tubes with your team information and clone designation (pgRNA#1 and pgRNA#2).
- Please note: you will add one of your candidate clone plasmid mini-preps to each tube and the pdCas9 to both tubes!
- Acquire an aliquot of the pdCas9 mini-prep (prepared by the teaching faculty) and of the competent MG1655 E. coli cells from the front laboratory bench.
- Pipet 100 μL of the MG1655 competent cells into each labeled eppendorf tube.
- Remember: it is important to keep the competent cells on ice! Also, avoid over pipetting and vortexing!
- Add 5 μL of the pdCas9 mini-prep to each tube.
- Add 5 μL of each pgRNA candidate clone mini-prep to the appropriate eppendorf tube.
- Incubate your co-transformation mixes on ice for 30 min.
- Start working on Part 3.
- Carry your ice bucket with your co-transformations to the heat block at the front laboratory bench.
- Be sure you also take your timer.
- Transfer the tubes with your co-transformations to the heat block set to 42 °C and incubate for exactly 45 sec.
- Remove your co-transformations from the heat block and immediately put them back in the ice bucket, then incubate for 2 min.
- Pipet 500 μL of pre-warmed SOC media into each co-transformation.
- Move your co-transformations to the 37 °C incubator and carefully place them on the nutator (secure your tubes by sliding them under the rubberband).
- Incubate co-transformations for 1 h.
- Retrieve your co-transformations from the incubator and alert the teaching faculty that you are ready to plate your samples.
- Plate 100μL of each co-transformation onto an appropriately labeled LB+Amp+Cam agar plate.
- The teaching faculty will demonstrate how you should 'spread' your co-transformation onto the LB+Amp+Cam agar plates. You should include this procedure in your laboratory notebook.
- Move your spread plates to the 37 °C incubator wherein they will incubate for ~18 hr.
Prepare media for mixed-acid fermentation inoculations
To test the effect of your gRNA on altering the production of either ethanol or lactate, you will incubate the co-transformed E. coli cells both with and without oxygen. Remember from lecture that cells grown anaerobically will produce more fermentation products; however, our goal is to further enhance the production of these products by manipulating the fermentation pathway.
- Acquire 4 glass culture tubes and 4 15 mL conical tubes from the front laboratory bench and label as shown below:
- MG1655 +O2 -aTc (glass tube)
- MG1655 -O2 -aTc (15 mL conical tube)
- MG1655 +O2 +aTc (glass tube)
- MG1655 -O2 +aTc (15 mL conical tube)
- MG1655 +CRISPRi +O2 -aTc (glass tube)
- MG1655 +CRISPRi -O2 -aTc (15 mL conical tube)
- MG1655 +CRISPRi +O2 +aTc (glass tube)
- MG1655 +CRISPRi -O2 +aTc (15 mL conical tube)
- Be sure to include your team information on each tube!
- Obtain a bottle of LB broth from the front laboratory bench.
- Transfer 5 mL of the media into each tube labeled for inoculation with MG1655 (alone, not co-transformed).
- Calculate the volume of the chloramphenicol and ampicillin antibiotic stocks that are required to maintain the pdCas9 and pgRNA plasmids, respectively, in the remaining volume of the LB media using the information below:
- For chloramphenicol: the stock concentration is 25 mg/mL and the final or 'working' concentration should be 25 μg/mL.
- For ampicillin: the stock concentration is 100 mg/mL and the final or 'working' concentration should be 100 μg/mL.
- Add the appropriate volume of each antibiotic stock to the LB aliquot and mix thoroughly.
- Transfer 5 mL of the media you prepared in Step #5 into each tube labeled for inoculation with MG1655 +CRISPRi.
On either Monday or Tuesday afternoon, depending on your laboratory section, the teaching faculty will inoculate MG1655 or a co-transformant into your culture tubes and add 2 μM aTc (final concentration) to the appropriate tubes. All tubes will then be incubated at 37 °C until you return for the next laboratory session.
Measure cell density of cultures
Before you prepare your samples to quantify the fermentation product yield, it is important that you measure the optical density (OD) of your cultures. This number will be used to normalize your data, which allows you to more accurately compare your samples. As a reminder, the OD is a measure of culture density and is based on the deflection of light at 600 nm (OD600). In this method of analysis, the higher the OD is the more dense the culture.
- Obtain an aliquot of fresh LB media and 9 plastic cuvettes from the front laboratory bench.
- Pipet 1000 μL of LB into 1 cuvette. This will serve as the blank needed to calibrate the spectrophotometer.
- Pipet 900 μL of LB into each of the 8 remaining cuvettes.
- It may be helpful to label your cuvettes with a simple designation that corresponds to your samples. Be sure that your labels are not in the light path as this will obscure your OD600 readings.
- Transfer 100 μL of each culture into the appropriate plastic cuvette.
- Note: this is a 1:10 dilution of your sample that should be accounted for in your calculations for product yield.
- Use the spectrophotometer to measure the OD600 values according to the instructions from the Orientation exercise.
- This step will be a bottleneck. Please be courteous to your laboratory mates and do not go to the spectrophotometer until your samples are fully prepared and ready to be measured.
Quantify fermentation product yield
Please be sure to follow the appropriate protocol below.
Measuring ethanol yield
Prepare supernatants from overnight cultures
- Transfer the cultures from your aerobic tubes to fresh 15 mL conical tubes.
- Be sure to label your tubes!
- Pellet the bacterial cells in your cultures by centrifugation using the large centrifuge on the teaching faculty laboratory bench.
- Alert the teaching faculty when you are ready for centrifugation.
- Centrifuge your samples at 3000 rpm for 10 min.
- If the media is still ‘cloudy’ repeat the centrifugation step.
- Pipet 1000 μL of the supernatant from each 15 mL conical tube into a fresh 1.5 mL eppendorf tube.
- Be sure to label your tubes!
- Label 8 additional eppendorf tubes and generate 1:15 dilutions of each of your samples in a final volume of 300 μL.
- Use fresh LB as the diluent.
Prepare samples for standard curve
- Prepare a 1 nmole/μL stock ethanol solution:
- In an eppendorf tube labeled A, combine 808.7 μL of the Ethanol Assay Buffer with 50 μL of the 17.15 N Ethanol Standard to generate a 1 μmole/μL solution.
- In an eppendorf tube labeled B, combine 990 μL of Ethanol Assay Buffer with 10 μL of solution A (from tube A) to generate a 10 nmole/μL solution.
- In an eppendorf tube labeled C, combine 900 μL of Ethanol Assay Buffer with 100 μL of solution B (from tube B) to generate a 1 nmole/μL stock ethanol solution.
- Label 5 eppendorf tubes as follows: 2 nmole, 4 nmole, 6 nmole, 8 nmole, and 10 nmole. (These will be the amounts in 50 μL samples, as will make sense in a few minutes.) Use the following steps to prepare samples for your standard curve that contain the specified amounts of ethanol.
- In the 2 nmole tube, combine 144 μL of the Ethanol Assay Buffer with 6 μL of the 1 nmole/μL stock ethanol solution you generated in Step #1.
- In the 4 nmole tube, combine 138 μL of the Ethanol Assay Buffer with 12 μL of the 1 nmole/μL stock ethanol solution you generated in Step #1.
- Prepare the 6 nmole, 8 nmole, and 10 nmole tubes.
- Note: the amount of ethanol in each tube is three times as much as specified by the label because you are preparing enough for duplicate samples (ie the contents of each tube will be divided between two wells in your assay resulting in the appropriate amount of ethanol in each well).
Complete ethanol assay
- You will prepare your assay plate according to the map above.
- Add 50 μL of the Ethanol Assay Buffer to wells A1 and B1. This is the 0 nmole/well sample for your standard curve.
- Add 50 μL of each of the remaining standard curve samples to the appropriate wells.
- Add 50 μL of your experimental samples according to the following order:
- To C1, D1 and E1 add MG1655 +O2 -aTc
- To C2, D2 and E2 add MG1655 -O2 -aTc
- To C3, D3 and E3 add MG1655 +O2 +aTc
- To C4, D4 and E4 add MG1655 -O2 +aTc
- To C5, D5 and E5 add MG1655 +CRISPRi +O2 -aTc
- To C6, D6 and E6 add MG1655 +CRISPRi -O2 -aTc
- To C7, D7 and E7 add MG1655 +CRISPRi +O2 +aTc
- To C8, D8 and E8 add MG1655 +CRISPRi -O2 +aTc
- Calculate the amount of Reaction Mix you will need for your assay using the following information:
- To account for pipetting error, you will add an additional 20% of each component to the mixture.
- You will add 50 μL of Reaction Mix to every well (supernatants and standard curve samples).
- The Reaction Mix consists of 46 μL of Ethanol Assay Buffer, 2 μL of Ethanol Probe, and 2 μL of Ethanol Enzyme Mix per 50 μL.
- Confirm your calculations with the teaching faculty before you proceed.
- Combine the appropriate amount of Ethanol Assay Buffer, Ethanol Probe, and Ethanol Enzyme Mix in a fresh, labeled conical tube.
- Add 50 μL of your Reaction Mix to each well in your 96-well plate that contains either standard or experimental samples.
- After each addition, use the pipet to mix the contents of the well.
- Be sure to change pipet tips between additions.
- Cover your 96-well plate tightly with aluminum foil and incubate at room temperature for 60 min.
- Alert the teaching faculty when your samples are ready for the spectrophotometer.
- Because the laboratory spectrophotometer is only able to measure a single sample in a cuvette, we will use a plate reader spectrophotometerto measure the A570 values for your assay.
Part 2: Analyze ethanol yield data
- Prepare a spreadsheet with the spectrophotometer values obtained for your assay.
- Average the technical replicates in your data set.
- Correct for the background ‘noise’ in your data by subtracting the averaged value of your 0 nmole/μL samples from the averaged values of all other samples.
- Plot the corrected values for your standard curve samples with the known concentration on the y-axis and the A570 (for ethanol) or A450 (for acetate) on the x-axis.
- Include the R-squared value and the equation of the best-fit line on your graph.
- Attach the graph to your Benchling laboratory notebook entry.
- Use the equation of the best-fit line to calculate the amount of fermentation produce in your experimental samples.
- For the ethanol and acetate assay: remember that you used a dilution of the supernatant in your assay!
- To calculate the concentration of product, use the following equation: Sa / Sv = C
- Sa = amount of fermentation product in unknown sample (nmole) from standard curve
- Sv = sample volume (μL) added to well
- C = concentration of fermentation product in sample
- The units of this calculation will be nmole/μL. For units of ng/μL use the molecular weight value of ethanol, which is 46.07 g/mol, or acetate, which is 60.05 g/mol.
- Lastly, normalize your data to the OD600 of your initial culture to account for difference in cell number.
- Divide the amount or concentration of fermentation produced by the OD value measured.
Reagents list
- Luria-Bertani (LB) broth: 1% tryptone, 0.5% yeast extract, and 1% NaCl
- chloramphenicol antibiotic stock (25 mg/mL)
- ampicillin antibiotic stock (100 mg/mL)
- anhydrotetracycline (aTc) stock (1 mM) (from Sigma Aldrich)
- Ethanol Assay Kit (from Sigma-Adrich)
- ethanol assay buffer
- ethanol probe
- ethanol enzyme
Next day: Examine genomic features in gRNA-targeted sequences